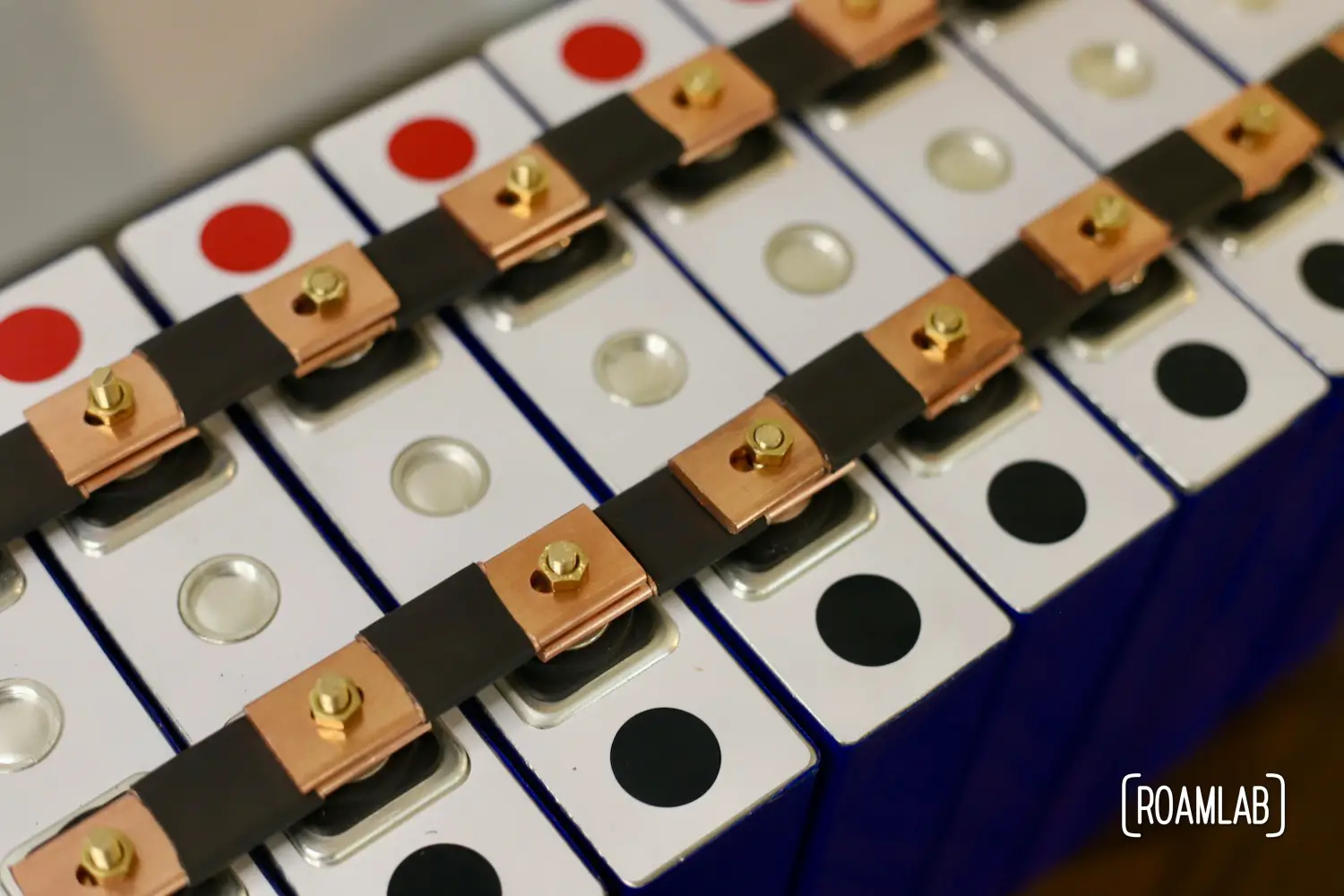
Parallel vs. Series: Connecting Cells To Build A Battery DIY Lithium Battery
Our truck camper may be half a century old, but we are installing a completely new electrical system. Rather than relying on propane, diesel, or other fuels, we are going all-electric with a solar power roof and a large battery bank. To get the most bang for our buck (or, more accurately, watts for our buck) we are building our own lithium battery. To this end, we bought sixteen 3.2V 180Ah LiFePO4 battery cells. But there are a lot of different ways we can arrange these cells. Here’s how we chose what to do.
Battery Cell Arrangements
When we talk about batteries, we generally speak in terms of a single, holistic power source. For small applications like cellphones, tablets, or clocks, one battery cell can do the trick. But for larger applications, that battery box tends to contain a collection of battery cells arranged to achieve a desired voltage and amperage. We can rearrange the same cells to produce a wide range of amps and volts. The mechanism determining the potential voltage or amperage of a cell array comes down to how the cells are connected: in series or parallel.
Parallel Connections
To connect a battery cell in parallel, we join all the positive terminals to each other and the negative terminals to each other. When we line up the cells like the image above, the positive and negative connections will create two parallel lines.
When we connect the cells in parallel, they add their amperage together, but the voltage stays the same. Therefore, two 3.2V 180 Ah battery cells linked in parallel would create a single 3.2V 360 Ah battery. In the case of diagram 1 shown above, we have four batteries in parallel, so:
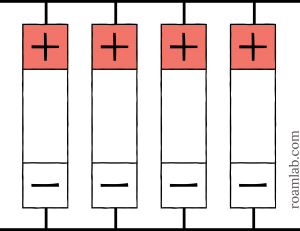
$latex 4\times 180Ah=720Ah&s=4 $
Series Connections
To connect a battery cell in series, we chain the positive and negative terminals of each battery. So, the negative terminal of the first cell connects to the positive terminal of the second cell. The negative terminal of the second cell then connects to the positive terminal of the third cell and so on.
By connecting cells in series, we are adding the voltage potential of each cell while the amp hours remain the same. For example, two 3.2V 180 Ah battery cells linked in series would create a single 6.4V 180 Ah battery. In the case of diagram 2 above, we have four batteries in series, so:
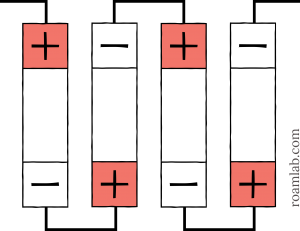
$latex 4\times 3.2V=12.8V&s=4 $
Rounding Voltages Up
Taking this one step further, what if we want a 12-volt system, as found in most RVs? We will want to connect four 3.2V cells to make a 12.8V battery as shown above. Notice that this isn’t exactly 12-volts. What matters here is that we achieve a voltage above what is needed to power the whole system. We don’t want to deviate too much, but the .8V in this case is completely acceptable. Most devices will have a listed tolerance for over-voltage. We can check that tolerance to make sure our batteries are within an acceptable range. In this case, whenever we refer to a 12, 24, or 48-volt LiFePO4 system, that technically means 12.8, 25.6, or 51.2 volts respectively.
Combining Series and Parallel Connections
Since a parallel connection will compound the amperage of a battery and a series connection will compound the voltage of a battery, we can arrange cells in combinations of series and parallel to achieve our desired voltage and amperage. Returning to our 12-volt example: we can connect four 3.2V 180Ah cells in series to create a 12.8V 180Ah battery. But what if we want a 12-volt battery AND more amp hours? We can increase the amp hour by adding cells in parallel to the existing arrangement. In this case, we can double the amperage by replacing the individual four cells in series with four sets of two cells in parallel and then wire those four parallel sets in series. Describing such an arrangement can quickly become confusing in sentence form. Fortunately, there are standard ways of expressing these complicated arrangements so that we can simply and easily explain the configuration of our cells.
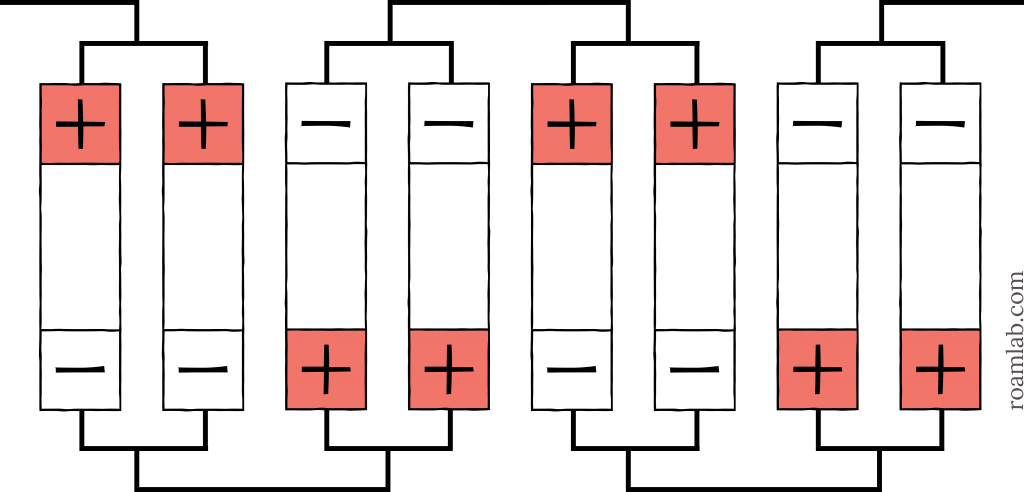
Cell Arrangement Nomenclature
Up until this point, we have mainly been discussing simple battery connections in either parallel or series. We can succinctly describe an arrangement of battery cells by labeling the number of cells followed by how they are connected: a “p” or “s” for parallel or series. A 4p configuration describes four cells in parallel. A 4s arrangement represents four cells in series.
As cell arrangements get more complicated, abbreviations can be combined to express the number, configuration, and order of the cells. Start with the base arrangement and work outward. If we have eight cells and split them up into sets of two, wired in parallel, and then connect those four sets in series, the result is a 2p4s arrangement, as shown in Diagram 3. That arrangement could even be one-third of a more extensive array in parallel: a 2p4s3p, as shown in Diagram 4. You can see how each additional collection of cells is effectively a multiplier.
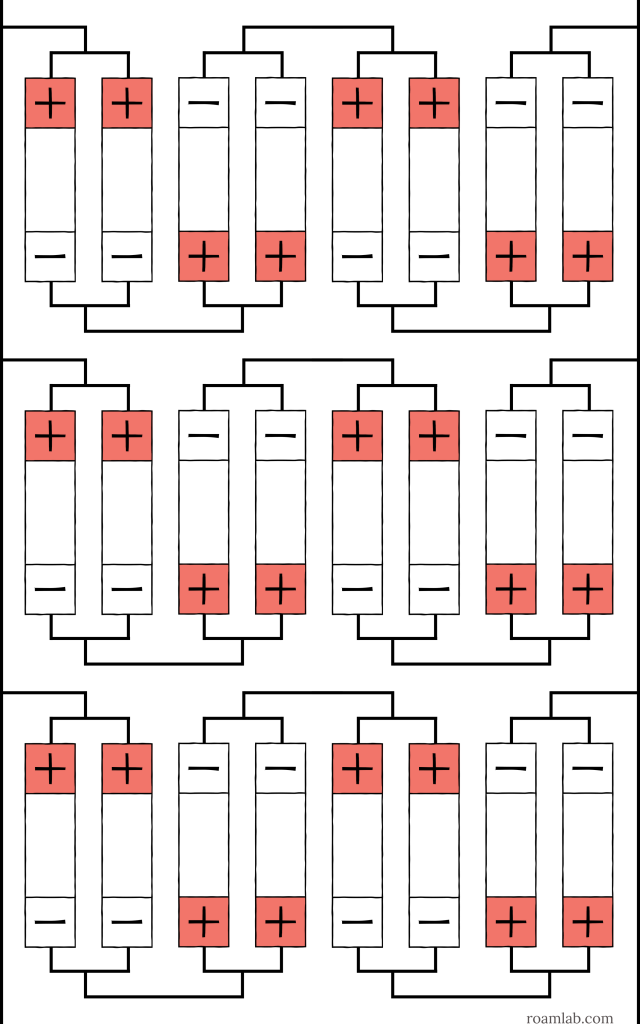
Our Practical Application
We have sixteen 3.2V 180Ah LiFePO4 battery cells at our disposal. That translates into 576 watts per cell or a total of 9216 watts. As we have discussed above, we can divide these cells into many different potential voltages and amperages. Depending on how we arrange the cells, we can have anywhere from a 3.2 to 48-volts. Taken another way, we could have a 180Ah battery to a 2880Ah battery. There are some logical reasons why we want to avoid these extremes, but they are still worth exploring for the sake of learning.
Arrangement | Volts | Amp Hours |
---|---|---|
16p | 3.2 | 2880 |
2s8p | 6.4 | 1440 |
4s4p | 12.8 | 720 |
8s2p | 25.6 | 360 |
16s | 51.2 | 180 |
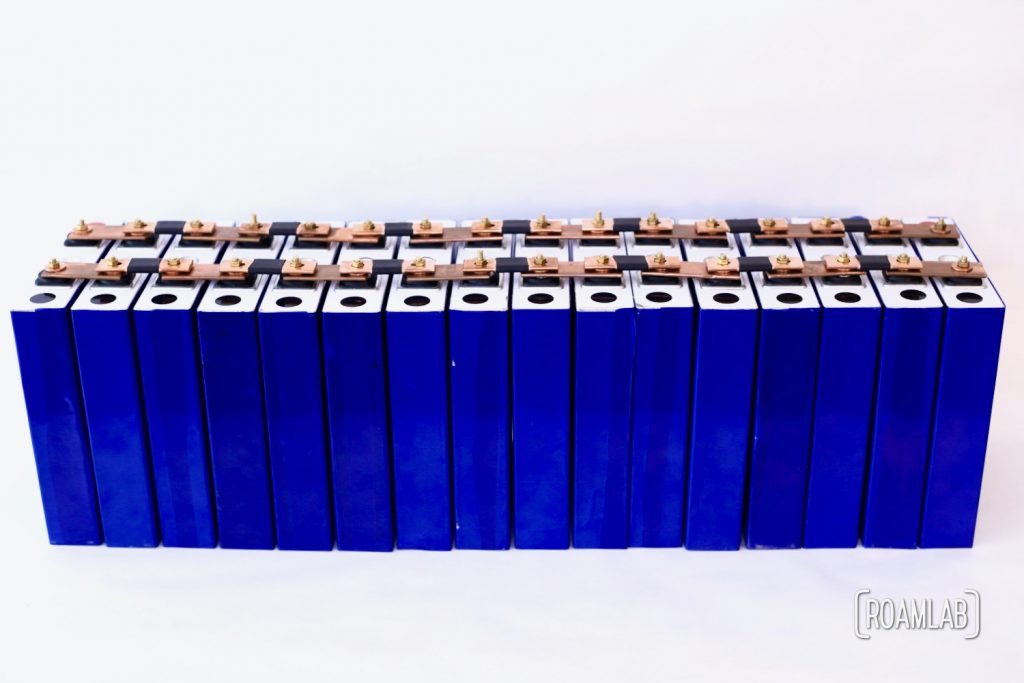
3 Volt System (3.2 V 2880Ah)
Usually, we will come in contact with 3-volt batteries in the form of coin cells to power our calculators, remotes, or other small hand held electronic items, not a whole RV. A 3.2-volt system doesn’t make much sense for our purposes. All the same, a hypothetical 3-volt system illustrates the maximum capacity of our battery array. Our battery cells are 3.2-volts. Therefore, the lowest voltage we can create with our cells is a 3.2-volt 16p battery. By arranging all the cells in parallel the voltage stays the same but the amperage is multiplied by the number of cells in the battery. That being the case, sixteen 180Ah cells in parallel will make a 2880Ah battery. Aside from there being limited applications for a 3-volt system, that is also a potentially dangerous amount of amperage.
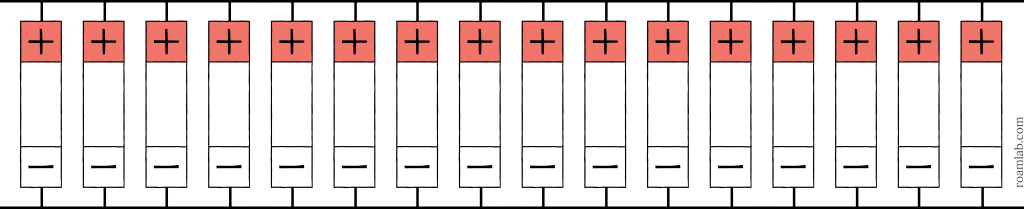
6 Volt System (6.4V 1440Ah)
Creating a 6-volt system is our first step into a series cell arrangement. To reach six volts, we need to double our voltage by splitting the cells in half so that we have eight parallel cells arranged in 2 series sets or an 8p2s arrangement. The eight parallel cells add up to 1440Ah and 3.2 V. Connecting these two sets in series compounds the voltage to create a single 6.4V 1440Ah battery.
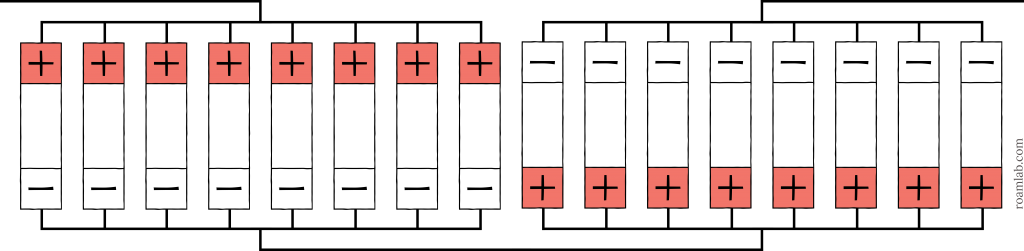
Different Arrangement, Same Result
It’s worth noting that we can flip this arrangement and create a 2s8p arrangement of batteries and have the same result. The battery will still produce 6.4 volts and 1440Ah, but we arrange the cells in sets of two series connections, and then all eight groups would be combined in parallel. The battery industry considers it best practice to arrange cells in parallel and then series, as seen in Diagram 6. This order makes the battery arrangement simpler to follow and decreases the likelihood of a mistake.
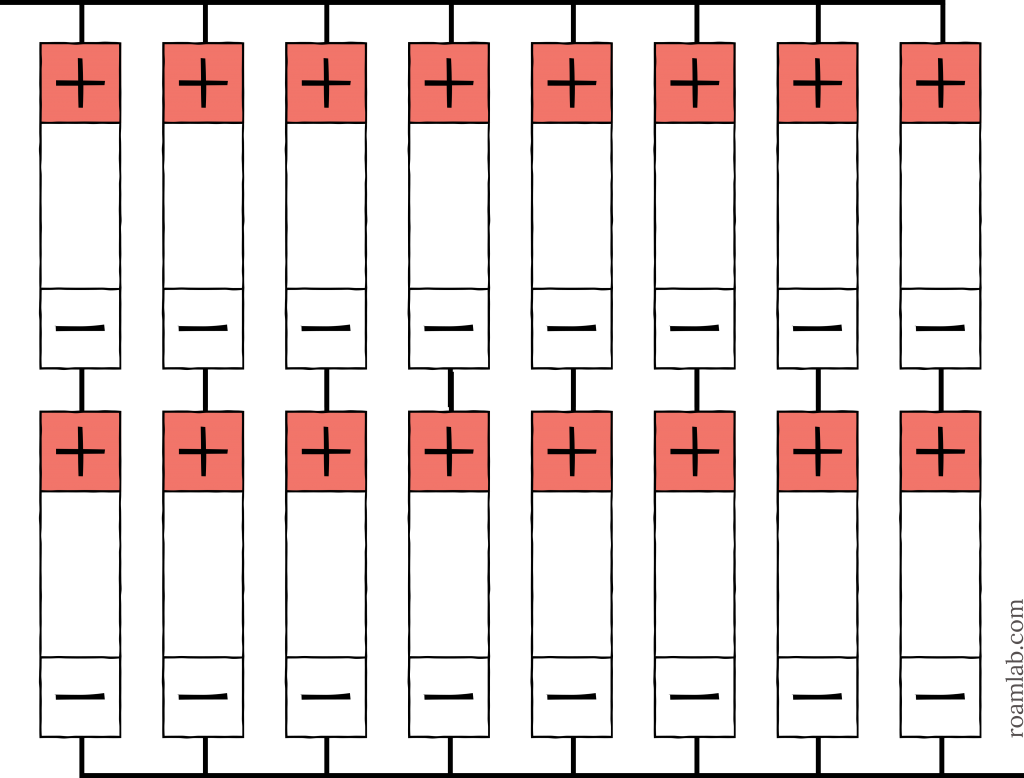
12 Volt System (12.8V 720Ah)
Once we reach 12 volts, we are starting to get into a more practical battery cell arrangement. 12-volt systems are a popular option for RVs. Most RV appliances are built for 12-volts. By arranging four 3.2V batteries in series, we have reached 12.8V, enough to power common RV appliances. With a collection of 16 battery cells, this means that we have a 4p4s arrangement. The four 180Ah batteries in parallel leave us with a 720Ah total system. While manageable, this is an amperage that is still expensive to have in an RV.
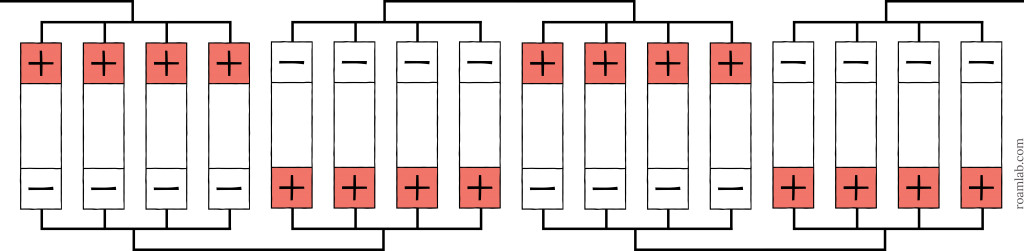
24 Volt System (25.6V 360Ah)
The 24-volt system is becoming a more popular option among RVers with a larger battery and solar system. The higher voltage means that high wattage systems rely on lower amperage and less expensive wiring. It takes eight 3.2V cells to reach 25.6V and supply enough volts to serve a 24-volt system. So, that means a 16 cell battery divided by eight (8s) splits our collection in half for a 2p arrangement. The resulting 2p8s cell arrangement leaves us with 360Ah capacity, which is much safer.
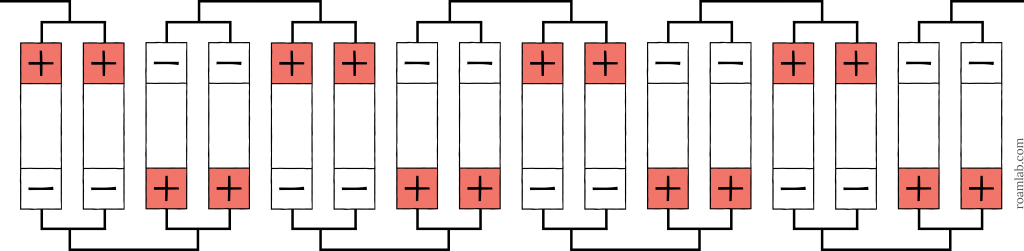
48 Volt System (51.2V 180Ah)
A 48-volt system is the upper limit of battery arrangements for campers. Any voltage 50-volts or below is considered “safe to touch.” Even so, 48-volts can deliver quite a shock. Regardless, a 48-volt system is the maximum voltage we can reach with 16 3.2V cells. Arranging them all in series, we achieve a 16s battery with 51.2 volts and 180Ah.
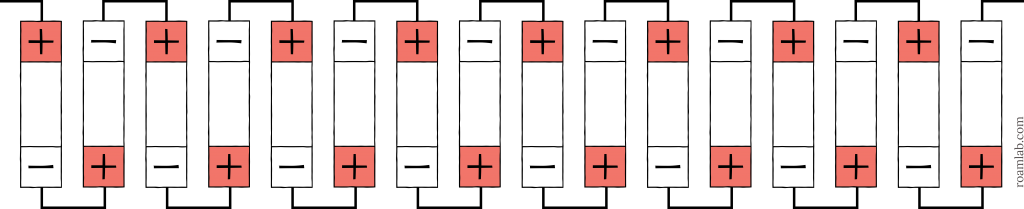
Our Setup
We settled on a 24-volt system. We gave the conventional 2p8s example in Diagram 9, but we are deviating from common practices to build an 8s2p battery. That means we will arrange 8 cells in series to reach 24-volts and then arrange those two series in parallel. While this is the industry standard of starting with a parallel arrangement, we have our reasons for deviation. Even so, we are following suit, in a way.
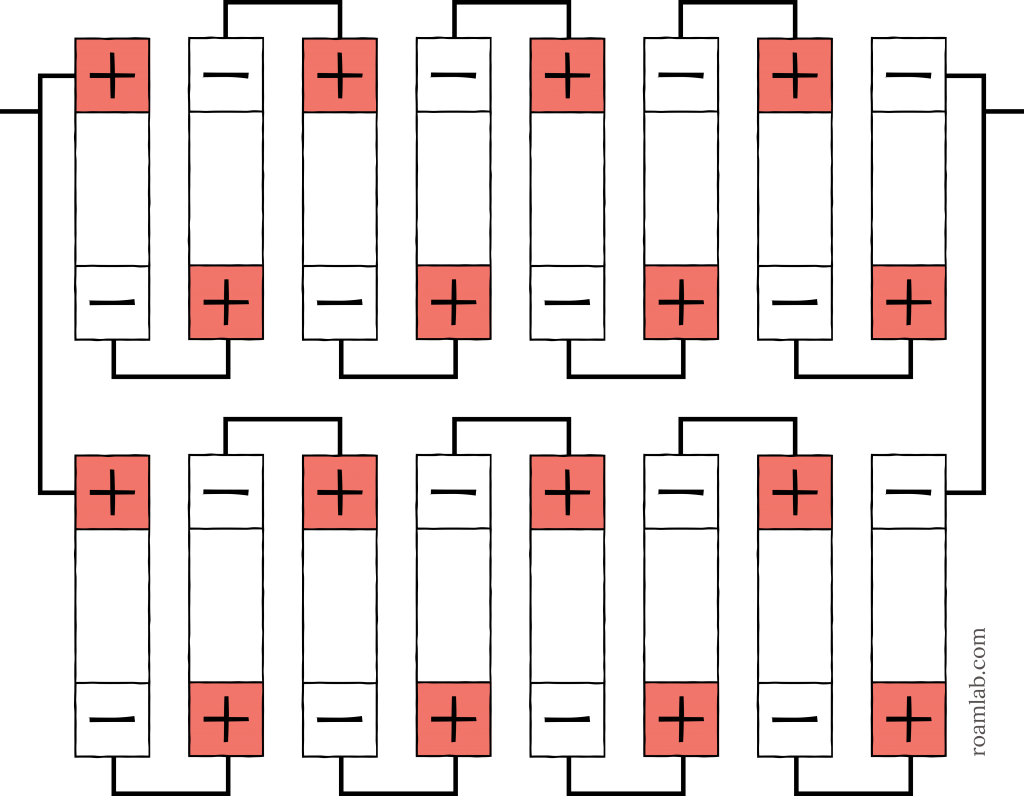
A 2p8s battery would be prohibitively heavy as one unit. While lithium batteries are known for how light they are, that is relative to lead-acid batteries. Each 3.2V 180Ah LiFePO4 battery cell weighs seven pounds. Individually, that isn’t much. But with sixteen cells, that makes for a total of 112 pounds. That weight is not including the battery box and accompanying hardware. Instead, we decide to split the cells into two sets with separate cases so that we can move them more easily. This arrangement means that we have two 8s (25.6V 180Ah) batteries, which we will arrange in parallel to have a 25.6V 360Ah system.
Now that we have defined what we are building, we can move forward: ordering a battery management system, inverter, and hookups to start charging the cells.